Research Signpost
37/661 (2), Fort
P.O., Trivandrum-695 023, Kerala, India
(Recent Res. Devel. Genet. Breeding,
2 (2005): 45-70 ISBN: 81-308-0007-1 )
Lönnig, W.-E.:
Mutation breeding, evolution,
and the law of recurrent variation.
In: Recent Research Developments
in Genetics and Breeding
(G. Pandalai, Managing Editor), Vol. 2 ,
45-70
(2005).
Wolf-Ekkehard Lönnig
Max-Planck-Institut for Plant Breeding Research, Carl-von-Linné-weg
10
50829 Cologne, Federal Republic of Germany
Correspondence/Reprint request: Dr. Wolf-Ekkehard Lönnig, Max-Planck-Institut
for Plant Breeding Research Carl-von-Linné-weg 10, 50829 Cologne,
Federal Republic of Germany. E-mail: loennig@mpiz-koeln.mpg.de
Abstract: Abstract In the present paper the history of the rise and fall of
mutation breeding as an autonomous branch of breeding research is documentedas
well as its positive side effects for plant breeding and biology in general.
Perhaps the most important generalization on the basis of the total outcome
of mutation breeding will be termed “the law of recurrent variation”.
It states that “treating homozygous lines with mutagenic agents
generates large, but clearly finite, spectra of mutants. This consistently
occurs when the experiments are carried out on a scale adequate to isolate
the potential of alleles causing phenotypic and functional deviations(saturation
mutagenesis). However, due to almost invisible residual effects of changes
in redundant sequences and/or of further chromosome rearrangements, the
corresponding saturation curve is asymptotically approaching its limit
for the micro-quantitative part of variation.” Also, reasons are
given why the law is relevant for heterozygotes and allogamous species
as well, and the genetical basis of the law is briefly defined.
In addition,
arguments are presented why the overoptimism and euphoria at the beginnings
of the period of mutation breeding are to be evaluated in connection
with the basic assumptions of the synthetic theory of evolution − i.e.
the assurance that mutations and selection constitute the entirely
sufficient explanation of the origin of all species and higher systematic
categories of the plant and animal kingdoms alike. This point established,
the question is discussed whether the finite nature of the mutant spectra
found in plant breeding research might also have repercussions on the
present theory of the origin of species.
Providing an affirmative answer of the
applicability of the law of recurrent variation not only to cultivated
plant and animal lines but also to species in the wild, the statements
and assertions of the synthetic theory as quoted below will have to
be revised. Introduction:
Hermann J. Muller, founder of mutation genetics and winner
of the “Nobel Prize in Physiology or Medicine” in 1946, summed
up the broad range of aspects and implications of mutation research in
his Nobel Lecture on “The Production of Mutations” (71).
Among several further topics, he discussed the question of teleological
tendencies in the process of variation itself (which he emphatically
denied), the proportions of lethal mutations to invisible and to visible
ones, also chromosome breakage and heterosis. One key point on mutations
in general certainly was his inference that due to the fact that “the
great majority of the changes should be harmful in their effects, just
as any alterations made blindly in a complicated apparatus are usually
detrimental to its proper functioning, many of the larger changes should
even be totally incompatible with the functioning of the whole, or, as
we say, lethal”. Hence, concerning medical aspects he later emphasized
that “it becomes an obligation for radiologists - though one far
too little observed as yet in most countries - to insist that the simple
precautions are taken which are necessary for shielding the gonads, whenever
people are exposed to such radiation, either in industry or in medical
practice”. Nevertheless, he was convinced that especially the ‘invisible
mutations’ (see below) were the basis of the origin of all life
forms, including man.
The pros and cons of two of the key elements of
mutation research, which Muller himself had regularly stressed before
and continued to do so after his Nobel Lecture, will be investigated
in the present paper: (a) the significance of this subject-matter for the
synthetic theory of evolution and (b), its applicability to animal and
plant breeding. For Muller, there was a clear connection between these
two topics, since his confident view of the potentials of mutations for
evolution provided the basis for the anticipated utilization in animal
and plant breeding. In fact, he was convinced “that for the first
time he had willfully changed the hereditary material and that evolution
could then be speeded up” (42). Thus, applying this evolutionary
hypothesis to breeding research, Muller himself stated that “for
the practical breeder, it is hoped that the method will ultimately prove
useful” (70), and likewise, that the production of mutations “may...prove
of increasing practical use in plant and animal improvement, in the service
of man” (71). Although for adaptation he favoured mutations “with
effects too small to have been detected at all by our rather crude methods”,
he thought that “under conditions of artificial breeding larger
mutations also can be nursed through to the point where they become suitably
buffered" − referring to Huxley’s idea on the method
how larger mutations could possibly be established in populations.
Also,
in the accompanying Nobel Presentation Speech by Professor T. Caspersson,
member of the Staff of Professors of the Royal Caroline Institute, this
idea was presented as follows (16):
“The extended knowledge of
the mechanism of the mutation processes has influenced and stimulated
the work in numerous fields outside theoretical genetics, and both theoretically
and practically important results have been reached. Merely to exemplify
the diversity and the varied nature of the spheres touched upon, I beg
to adduce a few examples: applied genetics, especially plant improvement,
which is of such practical importance, the theory of evolution, metabolic
research, a number of spheres within the realm of medicine, especially
perhaps eugenics and the theory of disease in general.”
And Muller
himself further stated the importance of mutations for plant breeding
and evolution in his Nobel lecture as follows (71):
“Not only is
this accumulation of many rare, mainly tiny changes the chief means of
artificial animal and plant improvement, but it is, even more, the way
in which natural evolution has occurred, under the guidance of natural
selection. Thus the Darwinian theory becomes implemented, and freed from
the accretions of directed variation and of Lamarckism that once encumbered
it.”
These statements of Muller and Caspersson should also be seen
on the background of a more general revolution in biology: the initiation
of the synthetic theory of evolution (neo-Darwinism), launched in 1937
by Dobzhansky’s book Genetics and the Origin of Species (22, 48).
From about that time on, the majority of evolutionists viewed mutations
(as defined by Muller) and selection to be the fully sufficient driving
force of the origin of all species and higher systematic categories of
the plant and animal kingdoms alike.
The essential part mutations play
in the synthetic theory was later summarized by Jacques Monod in the
following plain terms (69):
“We call these events [mutations]
accidental; we say they are random occurrences. And since they constitute
the only possible source of modification in the genetic text, itself
the sole repository of the organism's hereditary structures, it necessarily
follows that chance alone is at the source of every innovation, of all
creation in the biosphere. Pure chance, absolutely free but blind, at
the very root of the stupendous edifice of evolution: this central concept
of modern biology is no longer one among other possible or even conceivable
hypotheses. It is today the sole conceivable hypothesis, the only one
that squares with observed and tested fact. And nothing warrants the
supposition - or the hope - that on this score our position is likely
ever to be revised.”
The doyen of the synthetic theory, Ernst Mayr,
was of the widely accepted opinion that on the basis of mutations, “every
aspect of the "wonderful design" so admired by the natural
theologians could be explained by natural selection.” (63) And
Richard Dawkins, perhaps the most outspoken contemporary protagonist
of the theory, wholeheartedly assents in the following words (18):
“Never
were so many facts explained by so few assumptions [mutations and selection].
Not only does the Darwinian theory command superabundant power to explain.
Its economy in doing so [by mutations and selection] has a sinewy elegance,
a poetic beauty that outclasses even the most haunting of the world's
origin myths.”
For a documentation of many further voices principally
agreeing with these statements, see 17, 43, 44, 48, 62-64. Expectations
in mutation breeding
Since the origin of cultivated lines was thought
to be indispensably due to the same factors as the origin of species
in the wild, it has been reported that an enormous euphoria spread among
biologists in general and geneticists and breeders in particular that
the time had come to revolutionize the "old" method of recombination
breeding by the entirely new branch of mutation breeding (see documentation
below).
In other words: provided that mutations had, in fact, produced
the raw materials for the origin of all genes and proteins, all physiological
processes and anatomical structures of both the animal and plant kingdoms
alike, the most surprising successes had to be expected by applying these
factors − induced mutations and selection − to animal and
plant breeding research.
Also, three different time-lapse methods complementing
each other for a complete success in a rather short period of time
were at the disposal of the breeders: (a) multiplication of mutation
rates, (b) well-aimed recombination and (c) intelligent selection. Thus,
in the USA as well as in several countries of Europe and Asia, the new
research branch of mutation breeding was launched in what might be called
two waves: the first billow at the end of the 1930s, which was reinforced
especially after the Second World War to form a tide in cooperation with
the FAO/ IAEA, worldwide. Mutation breeding some 40 years later
In the following
paragraphs we will condense the general results for mutation breeding
after several decades of intense research of this branch by directly
quoting the authoritative statements of some of the world’s best
agronomical and botanical scientists, most of which have actively taken
part in mutation breeding themselves.
Thus, some 40 years after its beginnings
Simmonds sums up the inclusive results of the enterprise of mutation
breeding in his book on the Principles of Crop Improvement (84):
“Earlier
overoptimism, to the effect that induced mutations were about to revolutionize
plant breeding, has given place to a more sober appreciation of the technique
as a valuable supplement to more conventional techniques in certain,
rather restricted circumstances. ....[V]ery many programmes failed, especially
in the early days of overoptimism, to produce anything useful because
they were not fulfilled. Nowadays we see mutation-induction simply as
one technique which is occasionally useful in enlarging the genetic base
of a programme in a limited and highly specific fashion.”
Additionally,
Leibenguth describes the overall results of mutation breeding in his
work Züchtungsgenetik (Genetics of Breeding) as follows (40):
“Almost
all mutants distinguish themselves by negative selection values. According
to observations in cereals and legumes the proportion of mutants being
suitable for breeding amounted to 0.5 to 1 percent of the genotypes selected
in these experiments. Besides, often a negative effect on other components
of the pleiotropic spectrum of characters has been found that diminishes
the breeding value of a positive mutant. Thus, nowadays the original
aim to substitute the time-consuming and expensive methods of recombination
breeding by “mutation breeding” is not up-to-date anymore.
Mutation breedings is viewed to be less an autonomous method of breeding
than an occasionally used supplement to traditional methods.”
Already
some years before, Micke had stated that “one has to accept the
fact that only a very small fraction of induced mutants (certainly less
than 1 %) has ever been found suitable to enter yield trials and eventually
only 1 % of those evaluated passed the official tests and obtained approval
for commercial utilization” (67).
Over and above, Leibenguth adds
that mutation breeding cannot be successfully applied to animal husbandry
at all, because, “In contrast to plants, animals are genetically
more severely balanced. Hence, all kinds of mutations are even more frequently
lethal and more strongly diminishing vitality and fertility in animals” (40).
Hence, according to all the evidence achieved so far by experimental
investigations (and later also by careful considerations in theoretical
genetics) there is absolutely no future for mutation breeding in animals − not
to speak of severe ethical problems involved in the artificial mutagenesis
of birds, mammals and other animals capable of feeling pain.
In plant
breeding less than 1 percent of all the induced mutants have been chosen
as possible candidates for further investigations. Of these again only
0.5 to 1 percent have passed the necessary further field trials until
they were found suitable for commercial use. Thus, in plant breeding
the average proportion of negative or useless mutants to positive ones
is smaller than 10,000 : 1. Making calculations on the basis that only
0.5 percent of all induced mutation were suitable for further investigations
and that again only 0.5 percent displayed a positive selection value
for the breeder, this proportion is about 40,000 : 1. An approximate
mean value of 25,000 negative (or useless) mutants to 1 being positive
should therefore not to be an unrealistic calculation for plant breeding.
As to the genetically even more severely balanced animals, the state
of affairs has been so arduous that no realistic numbers have been produced,
which could provide the basis of similarly approximate calculations regarding
the proportions of negative (or useless) mutants to positive ones in
animal husbandry. If − as an educated guess − one multiplies
the proportionate number of disadvantageous mutations by the factor of
10, the result would already be some 100,000 to 400,000 negative (or
unavailing or neutral) mutants to 1 useful for breeding research.
It
was on the basis of such experiences often made over dozens of years
that almost all commercial breeding stations in the USA and Europe have
deleted mutation breeding from their research programmes.
A significant
concrete example may back up this point: at the end of the 1960s it was
still widely believed that it was possible to improve crop proteins by
mutation breeding. After some one and a half decades of intensive efforts
and extraordinary financial input, Micke and Weindl comment (68):
“Our
programme on the improvement of grain protein has now come to an end.
...[D]uring the 14 years of the programme it had to be recognized that
the matter is more complicated and that there are some mutual limitations
of quantity and quality.”
Poehlmann has summed up the overall results
of mutation breeding in agreement with the authors quoted above as follows
(76):
“One can only conclude that the results from mutation breeding
in varietal development of the major field crops have been rather meager
in relation to the efforts expended.”
Peter von Sengbusch concurs
by the following observation (82):
“In spite of an enormous financial
expenditure, the attempt to cultivate increasingly productive varieties
by irradiation, widely proved to be a failure.”
Also, the distinguished
plant breeders Fischbeck, Röbbelen and Stutzer are in accord with
these statements (25):
“The objectives of practical plant breeding,
to achieve new opportunities of a gradual and continuous amelioration of
tried and tested breeding varieties could...not be realized.”
And
especially concerning the neo-Darwinian concept of “micro-mutations” these
three authors continue (25):
“Also, the modified concept of a direct
use of so-called “micro-mutations” remained unsuccessful, because
achievable breeding progress by this method distinctly lagged behind useful
variation, which could be developed from the broad stream of conventional
recombination breeding.”
Yet, perhaps one of the most astounding
facts in the history of genetics appears to be the enormous gulf between
the optimistic descriptions of mutants by so many authors active in plant
breeding research during that period of time and the later "widely
spread disappointment regarding mutation breeding" (66) due to the
disconcerting reality, i.e. the meagre results obtained. Confirming the
observation of a rather strange distance between hypotheses and reality,
Micke continues his assessment after his calculations quoted above (explaining
the relatively few useful mutants achieved in mutation breeding) as follows
(67):
“In contrast to such rare achievements there have been innumerable
'promising mutants' reported in innumerable publications, which never seem
to appear again on the stage after their first presentation. Nevertheless,
there remains a respectable number of mutants which even the self-critical
breeder or geneticist have seriously considered as progressive and of which
only very few so far have contributed to the development of better crop
cultivars.
This experience has been disappointing to many, to those who
worked with mutations and expected optimistically fast 'break-throughs'
as also to those who watched the many mutation activities sceptically
but nevertheless hoped that results would make the difficult task of
plant breeders easier, at least in particular areas.”
Micke also pointed
out that neither the application of different mutagenic agents, nor various
degrees of dosages, nor diverse modifying measures were able to revise
the overall results: "The ultimate hope of obtaining more of the 'better'
mutants has not been fulfilled" (67) (see also note 1 at the end of
the paper).
Synopsis
According to the premises of the synthetic theory,
explaining the origin of the entire world of organisms predominantly
by selected mutations, a worldwide revolution in plant breeding research
had been expected in the late 1930s, which was reinforced by Nobel
laureate Josef H. Muller in 1946 especially for first decades after the
Second World War.
However, due to the fact that:
(a) “many programmes
failed...to produce anything useful”,
(b) “almost all mutants
distinguish themselves by negative selection values”,
(c) “all
kinds of mutations are even more frequently lethal and more strongly
diminishing vitality and fertility in animals”,
(d) the overall
results “have been rather meager in relation to the efforts expended”,
(e) “in spite of an enormous financial expenditure... [mutation
breeding] widely proved to be a failure”,
(f) “the objective
of practical plant breeding...could not be realized” neither by “macro-mutations” nor
by “micro-mutations”,
(g) none of the modifying measures
applied could help fulfilling “the ultimate hope of obtaining more
of the ‘better’ mutants”,
- the overall result was
that these strong anticipations concerning a revolution
in plant breeding, accompanied by an intense euphoria especially among
geneticists and agronomical scientists after the Second World War, ended
up in a worldwide failure and breakdown of mutation breeding as an autonomous
branch of breeding research in the 1980s at the latest in most Western
countries.
The status
of mutation breeding today is that of “an occasionally used supplement
to traditional methods”, just “occasionally useful in enlarging
the genetic base of a programme in a limited and highly specific fashion”.
To answer the question, what this “limited and highly specific
fashion’ could essentially consist of, one should be aware of the
fact, that mutations usually produce weaker or non-functional alleles
of wild-type genes. Such mutagenic effects can be useful in plant breeding
research when, for example, some of a plant’s secondary metabolites
are disadvantageous for human consumption. If the gene functions necessary
to produce such metabolites can be switched off by mutations without
greater pleiotropic shortcomings for the plant as a whole, such a mutant
could be interesting for further breeding.
In fact, Reinhold von Sengbusch
(the father of Peter), perhaps Germany’s most successful plant
breeder of the 20th century, summed up the essence of plant breeding
by stating that − apart from polyploidy − the transformation
from the wild to the cultivated plant is genetically characterized mainly
by the fact that the features of the wild plants are dominant and those
of the cultivated lines are recessive (83). Usually, recessiveness means
losses of gene functions (for a documentation, see 43). As inactivations
are the most common effect, which ‘normal’ mutations and/or
mutations generated by transposons are exerting on genes (thus producing
recessive alleles), the inference can be made that − as far as
gene inactivations are important for breeding − mutations might “occasionally” still
be relevant for some further progress (see also some reviews on transposons,
where these points are further discussed: 1, 2, 10, 11, 38, 43, 54 -
56).
Yet, in our age of molecular genetics, tools are being developed
that should increasingly allow directed mutagenesis to inactivate genes
coding for undesirable second plant metabolites thus substituting conventional
mutation breeding by accidental mutations probably entirely in the near
future.
Notwithstanding, the enormous efforts of mutation breeding had
also some unequivocally positive side effects for plant breeding research
in particular and basic research of biology in general, which will
be the topic of our next paragraphs.
Some positive “side effects” of
mutation breeding
Although the enormous successes and world-wide revolution
firmly expected in plant and animal breeding in connection with the assumptions
of the synthetic theory did not materialize, science nevertheless profited
from the intense efforts of mutation breeding “by a rapid increase
of the information on the localization of genetic effects in the genome
of important cultivated plants” (25).
Thus, basic scientific research
has substantially benefited from this enterprise. In other words: “Although
the production of plant mutants was economically unprofitable, it probably
proved to be the most effective experimental instrument of modern basic
research” (82).
I have called the most important result of this
branch of basic scientific research fully relevant for both, the origin
of species and for mutation breeding, the law of recurrent variation
(45) (see also note 2 at the end of the paper), which will be the topic
of the next paragraphs.
Deducing the law of recurrrent variation
An essential
experimental discovery supporting the improbability of the origin of
all life forms due to mutation, recombination and selection alone is
the fact (well-known for decades) that − after repetitive mutagenic
treatment of all the lines and species tested so far − the spectrum
of mutants will only slightly be increased. In other words, there is
a regularity in the appearance of the overall mutant phenotypes. After
40 years of intensive mutation research in Antirrhinum no less a geneticist
than Hans Stubbe has summed up his studies as follows (87):
“The
continually improved knowledge of mutants in Antirrhinum has provided
some essential experience [or results]. During the years each new large
mutation trial showed that the number of really new mutants recognized
for the first time, was steadily diminishing, so that the majority of
the genetic changes was already known.”
Similarly, Werner Gottschalk,
another of the world’s leading mutation geneticists stated (28):
“The
larger the mutant collections are, the more difficult it is to extend
them by new mutation types. Mutants preferentially arise that already
exist.”
In other words, the number of mutants with new phenotypes
asymptotically approaches a saturation line in persistently large mutation
experiments.
The results of mutation breeding in barley achieved by Udda
Lundqvist from the breeding station in Svalöf (Sweden) in decades
of experiments will clearly illustrate the phenomenon of recurrently
appearing mutants. She reported at the end of the 1980s that during the
last 50 years about 9,000 barley mutants have been isolated. Including
lethal mutants, there were identified at least 100,000 mutants (59).
The following examples of Table 1 of the recurrent appearance of special
types of mutants have been compiled from a paper of Lundqvist (58):
Table
1. Examples of repetitive appearance of certain types of barley mutants
compiled according to data published by Lundquist.
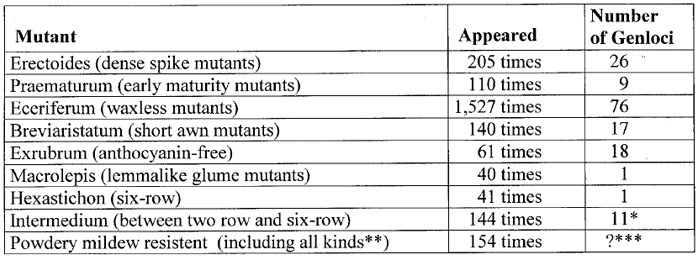
*103 of these cases investigated on 11 int gene loci.
**77 mutants
were resistant against race D1, 48 had complete resistance, and 29 displayed
brown necrosis.
***Of 72 investigated resistant mutants, 54 were found
to be distributed on 8 genes (the 28 recessive mutants belong to one
single locus); for the remaining 18 mutants the number of loci does not
appear to be fully established.
Thus, these 9 types of mutants have appeared
altogether 2,422 times. According to Lundqvist the 9,000 barley mutants
isolated during some 50 years of extensive mutagenesis experiments, could
be grouped into exactly 93 distinguishable types or classes (see Table
2). Many of these mutant types have appeared more than 100 times, some
even more than 1,000 times.
Closely comparable results have been achieved
for all other crops, which were included in mutation breeding research − as
for examples, the pea (Pisum sativum), rice (Oryza sativa), corn (Zea
mays), soybeen (Glycine max) and many others (43).
Table 2. Types of mutants in barley (according to
Lundqvist (60); numbers in brackets added by W.-E.L).
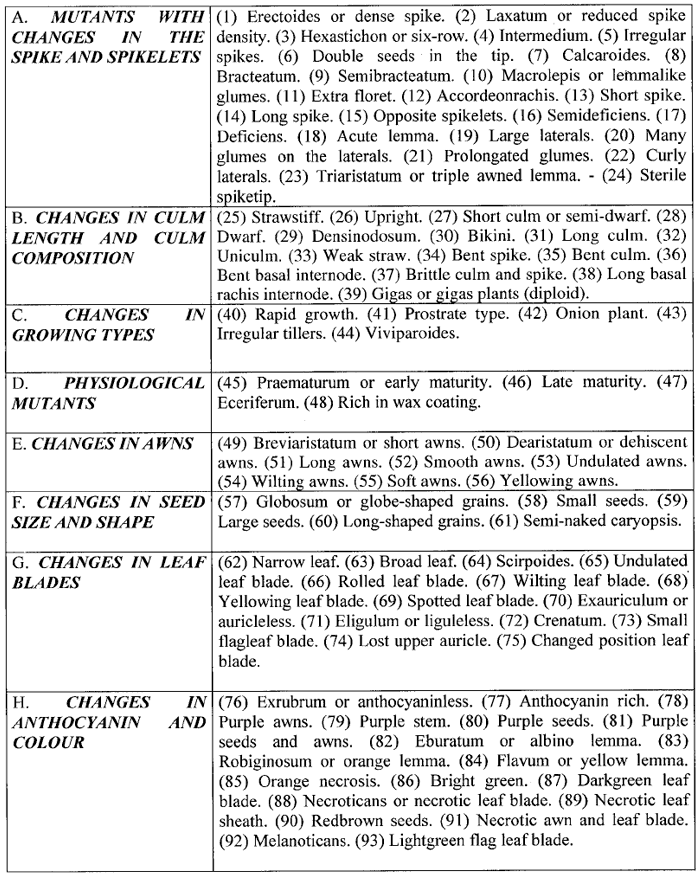
Taking the work of all research stations and breeding companies around
the world together, probably millions of barley mutants were induced.
Of these only 25 were found to be acceptable for the list of commercial
barley cultivars and 33 were added due to recombination (34). However,
since the average cultivation time of a commercial barley line is only
about 10 years, most of these lines will not be cultivated any more.
Although “mutants
for just about every recognizable trait exist” (34), the results
in barley may clearly illustrate Poehlmann’s comment, that, as quoted
above, “One can only conclude that the results from mutation breeding
in varietal development of the major field crops have been rather meager
in relation to the efforts expended” (76).
It may also be pointed
out in this connection that − as far as the author is aware − neither
plant breeders nor geneticists have ever reported the origin of any new
species, or just any new stable races or ecotypes either surviving better
or at least as well in the wild in comparison with the wild-type, in which
the mutation(s) have been induced (43, 45, 52, 55).
As to the recurrently
appearing mutants, two pioneers of plant breeding research, Kuckuck and
Mudra, emphasized the following key point already in the midst of the
last century (37):
“As extensive experiments have shown especially in
barley, the entire array of lines of the world seed bank (Weltsortiment)
can be mutatively induced by X-rays. ...In part, these induced mutants
proved to be genetically identical with similar lines of the world seed
bank. In other cases the same phenotypes are due to different genes; thus
in the latter cases so-called heterogeneous groups of features have been
detected.”
Similar observations have been reported for several other
crop plants as rice and maize. Instead of the regular and perpetual formation
of new useful culture varieties, subspecies and species, incessantly
the same spectrum of mutants is reproduced, so that after a certain number
of experiments the method is hardly useful for plant breeding anymore.
My own studies during the last 40 years (including experiments with
Pisum, Antirrhinum, and Misopates - altogether more than 2 million plants
investigated) are in full agreement with the results of the authors just
cited (38, 43-57, 81).
To comprehend and interpret these observations
correctly one must clearly distinguish between the two levels of investigations
in genetics: first, the level of the phenotypes, and second, the
DNA level. On the latter, the potential of missense and nonsense mutations
and other sequence deviations is nearly infinite. However, the spectrum
of the resulting different phenotypes is not, because the space of
functionally valid sequences within a given system of tightly matching
regulatory and target genes and correspondingly co-ordinated functions
involved in the formation of the finely balanced whole of an organism,
cannot infinitely be stretched by chance mutations.
To take a rather crude illustration
(see also (44) exemplifying Muller’s
case of an apparatus as quoted in the introduction): Drop your computer
from the desk or take a screwdriver and a hammer, open the casing, shut
your eyes and then forcefully operate in the innards! Depending on the
number of computers and how often and for how long one proceeds to act
this way, one may collect a nearly endless number of non-functional changes.
Yet - with much luck - one may also select a few operationally diminished,
but nevertheless still working, systems. Thus, one may demolish a computer
in a thousand and more different ways by some accidental procedures.
However, the resulting still more or less functional states (the functional
phenotypes), will be limited. The hope to secure a Pentium V from a
486er by this method would be very bold indeed. - Of course, the situation
in biology is more complex than in engineering, because organisms are,
for instance, not only passive, but also reactive entities. Nevertheless,
limits to selection have repeatedly been found in several areas of biological
research.
Figure 1. Idealized saturation curves illustrating
decrease of the number of new mutant phenotypes and new mutant genes
in increased number of experiments until saturation limits are reached.
Abscissa: increasing number of mutation experiments. Ordinate: (a) number
of mutant phenotypes, and (b) number of mutations (DNA-level) with effects
on the phenotype. Because mutations at different loci can cause similar
or identical phenotypes (see Table 1 above), the curve for the number
of mutant genes is distinct from that of the number of new phenotypes.
The redundancy problem - for example, some phenotypes appear only when
2 or more genes have been mutated (see further points in the text) -
widens the distance between the two curves. The real curves will be different
for different organisms, depending, among other things, on the genetic
complexity of the species involved as well as on the scale and specificity
of the experiments realized (different kinds and quantities of radiation,
chemical mutagens, transposons, t-DNA). The common ground of all curves
is the finite number of mutant phenotypes and mutant genes with effects
on the phenotype (apart from a micro-quantitative rest of variations
due to, for example, environmental and epigenetic factors, position effects,
and ‘junk DNA’, which, however, does not change the basic
situation).
The aptness of Muller’s comparison of mutations with
accidental changes in a complex apparatus has been reinforced by molecular
genetics during the last decades. Behe has recently recapitulated the
point for a larger audience as follows (6):
“The resemblance of
parts of life to engineered mechanisms like a watch is enormously stronger
than what Reverend Paley imagined. In the past 50 years modern science
has shown that the cell, the very foundation of life, is run by machines
made of molecules. There are little molecular trucks in the cell to ferry
supplies, little outboard motors to push a cell through liquid.
In 1998
an issue of the journal Cell was devoted to molecular machines, with
articles like ''The Cell as a Collection of Protein Machines'' and ''Mechanical
Devices of the Spliceosome: Motors, Clocks, Springs and Things.'' Referring
to his student days in the 1960's, Bruce Alberts, president of the National
Academy of Sciences, wrote that ''the chemistry that makes life possible
is much more elaborate and sophisticated than anything we students had
ever considered.'' In fact, Dr. Alberts remarked, the entire cell can
be viewed as a factory with an elaborate network of interlocking assembly
lines, each of which is composed of a set of large protein machines.
He emphasized that the term machine was not some fuzzy analogy; it was
meant literally.” Additionally, the observation that none of the
different methods of mutagenesis − from delicate experiments looking
for optimal mutation frequencies in plant breeding to the most massive
mutation inductions − have ever changed the fact of selection limits
(detected for all the plant and animal species so far investigated),
is in agreement with the facts just mentioned as well as with the saturation
curves shown above (43).
One of the best contemporary population geneticists,
Daniel L. Hartl, has summed up the question of selection limits as follows
(32):
“Progress under artificial selection cannot go on forever,
of course. As noted earlier, the population will eventually reach a selection
limit, or plateau, after which it will no longer respond to selection.
...However, many experimental populations that have reached a selection
limit readily respond to reverse selection.”
And some years later
Hartl und Jones have emphasized this empirical fact again (33):
“Population
improvement by means of artificial selection cannot continue indefinitely.
A population may respond to selection until its mean is many standard
deviations different from the mean of the original population, but eventually
the population reaches a selection limit at which successive generations
show no further improvement.” Both, in the animal and plant kingdoms, selection limits have been
detected, which could not be overcome in spite of persistently intensified
mutagenesis. The basic reason is that the spectrum of mutant phenotypes
is large but nevertheless limited. Moreover, this gamut of recurrently
emerging large yet finite numbers of different mutants is reproducible
as many times as correspondingly large populations are repeatedly mutagenized
and investigated.
Considering the safe predictability, regularity and
reproducibility of the phenomenon, we might thus formulate the law
of recurrent variation as shown in the next paragraphs. Formulating the
law of recurrent variation
In agreement with the facts referred to above
we can formulate the law of recurrent variation as follows:
Treating
homozygous lines with mutagenic agents generates large, but clearly
finite, spectra of mutants. This consistently occurs when the experiments
are carried out on a scale adequate to isolate the potential of alleles
causing phenotypic and functional deviations (saturation mutagenesis).
However, due to almost invisible residual effects of changes in redundant
sequences and/or of further chromosome rearrangements, the corresponding
saturation curve is asymptotically approaching its limit for the micro-quantitative
part of variation.
Because mutations at different loci often cause
similar or identical phenotypes (most traits are polygenic), the curve
asymptotically approaching the saturation line for the mutant genes
is distinct from that of the mutant phenotypes. In absolute terms, the
distance between the curves is also aggravated by the redundancy of (1)
the genetic code, (2) of gene functions, and (3) of conservative amino
acid substitutions, allowing mutant proteins to approximately fulfill
their original tasks and functions.
However, researchers must constantly
remind themselves that the law of recurrent variation focuses only
on DNA variations displaying measurable effects on the phenotype and
usually disregards the rest (see also transposons, below).
In practice
this means that in repetitive mutagenesis experiments the number of
new mutants differing phenotypically from each other is steadily diminishing
until a saturation limit is reached and progress under artificial selection
comes to an end. Given similar genetical
preconditions, the spontaneous mutation process in the wild will produce
the same large but limited spectra of mutants, which have appeared in
mutagenesis experiments. Yet, due to the decidedly lower mutation rate
under natural conditions, much larger populations are needed to realize
that potential − apart from the fact that most of the mutants will
disappear shortly after their arrival because of their negative selection
values.
Also, the law is valid for heterozygous lines and allogamous species.
However, due to uninterrupted gene flow the curves will approach
the saturation limits more slowly than in homozygous lines and autogamous
species.
As for a discussion of the micro-quantitative part of variation,
see (45). The genetic basis of the law of recurrent variation
The
genetic reasons for the law are rather simple. There are only a limited
number of genes, which, upon mutation, can produce a restricted number
of alleles. Stig Blixt has summed up the point from the plant breeder’s
view as follows (14):
“The number of gene combinations possible
to form from ten thousand genes is infinite. If the plant breeder
had to consider the entire gene-material as his field of work, the
question posed ["...whether precise controlled plant breeding
is a realistic possibility..."] would have to answered in the
negative. This, however, is not the case.
A large − probably
the major − part of the genes functioning in a plant are most
certainly of no concern for the plant breeder, inasmuch as, being
essential for the basic function of the cell and differentiation
mechanisms, all genetic variation in such genes represent 'forbidden
mutations', in the sense that the resulting mutants are completely
non-functioning and immediately eliminated. A certain part of the
genome may thus exist in probably one specific combination only,
representing what may be called the ultra-conservative part of the
system. Another large part of the genome, which may then be called
the conservative part, seems, although less rigidly, still to be
required to be present in the developed form to produce a 'normal'
organism able to carry out the plant functions in such a way as to
result in a reproductive, competitive individual. Mutations in this
part of the genome result in lethals, in sterile individuals, maybe
in different kinds of severe chlorophyll deficiencies, and so on.
Thus what has to be dealt with is certainly not the entire genome,
but only a part of it, the variable or redundant part, and in all
probability this constitutes a minor part. Even if the number of
genes may still amount to thousands, this is a restricted and finite
number, which will, moreover, also in progenies from crosses, contain
homozygous blocks of varying size.”
As to the statement that, “what
has to be dealt with, is certainly not the entire genome, but only
a part of it, the variable or redundant part, and in all probability
this constitutes a minor part” − the question of the
biological meaning of transposable elements (TEs) might be raised,
since up to about 90 percent of a plant’s genome can consist
of different families and classes of TEs. These problems have been
extensively discussed in 1, 2, 38, 43, 54-56, 86. If the hypothesis
is correct that most TE multiplications constitute weakly parasitic
events without doing too much harm for the lines and species thus
affected, this relatively huge DNA mass might be largely irrelevant
for the plant breeder in the field (there are, however, several pertinent
TE-effects, which appear to be similar to normal mutations like the
production of alleles, including total inactivations of genes, and
possibly some further ones not to be discussed here).
Nonetheless,
since the DNA mass (pg) can vary strongly between closely related
forms (species of the genus Vicia, for instance, vary between 1.8
and 13.3 pg per haploid genome (72, 74, 75), and because even within
the same non-polyploid plant species, the C-value can vary substantially
(8, 9) − generally
without known serious effects relevant for the plant breeder − we
might conclude that the assessment of Blixt quoted above, is essentially
still correct for any practical purposes even in our age of molecular
genetics (for further details see 1, 2, 10, 38, 55, 56). So focusing
on that variable or redundant part of the genome, in which mutations
induce phenotypically deviant, but still rather viable, competitive,
and fertile individuals and lines - what kind of variations do we
detect upon closer inspection as to quality?
The variations induced
are mostly losses-of-function-mutations (often including many alleles
with a series of gradually reduced functions) and they are either
neutral or slightly disadvantageous for the organisms thus affected,
yet in some cases they are useful for the breeder as well as micro-evolution
as shown above. Generally, “the great majority of the changes
should be harmful in their effects, just as any alterations made
blindly in a complicated apparatus are usually detrimental to its
proper functioning...” (see Muller, as quoted in the introduction). However, what has never been achieved by accidental mutations, is
the creation of entirely new functional DNA-sequences constituting
new genes and new gene reaction chains for novel synorganized anatomical
structures and/or physiological functions. Thus, in accord with
the laws of probability, examples and cases relativizing the law
of recurrent variation have not been observed so far (35, 43, 46,
65, 77, 78, 88, 95, see also note 2). In fact, also all the models
and data recently advanced to solve the problem of completely new
functional sequences and the origin of new organs and organ systems
by random mutations proved to be grossly insufficient upon close
inspection and careful scientific examination (for the details, see
12, 13, 27, 36, 41, 73, 90, 91). Corroboration of the law of recurrent variation by Vavilov’s
law of homologous series in variation
On the basis of comparable
variations in related species, genera and families in the plant and
animal kingdoms, the Russian geneticist and agrobiologist Nikolaj
I. Vavilov has formulated the law of homologous series in variation
(92, 93). He was able to trace back the beginnings of the law's detection
to the opponent of Darwin’s theory, Mivart 1871.
Vavilov has
summed up the law as follows (93): “Species and genera that are genetically closely related are
characterised by similar series of heritable variations with such
regularity that knowing the series of forms within the limits of
one species, we can predict the occurrence of parallel forms in other
species and genera. The more closely related the species...in the
general system, the more resemblance will there be in the series
of variations.”
“Whole families of plants in general
are characterized by definite cycles of variability occurring through
all genera and species making up the family.” As to the parallel
variations, Haecker (31) commented that they [mostly] appear to
be nonessential phenotypic deviations of the species thus affected.
Otherwise, he argued, it would be incomprehensible why these variations
should be connected with the strongly different types of clearly
defined species, genera and families. He also noted that the more
widely occurring parallel variations seem to be either due to mutant
losses of functions, or display a slightly degenerative to biologically
indifferent character without tightly correlative connections to
the species as a whole.
The most important inference of Vaviliov's
law corroborating the law of recurrent variation consists of the
fact that the parallel series of recurrent mutant phenotypes − generally
including closely related forms − do not level out the essential
species and genera differences. Otherwise it should be possible to
mutationally transform one species or genus into another closely
related one. However, nobody has ever reported something like the
morphological transformation of a pea (Pisum sativum) into a fertile
grass pea (Latyrus sativus) (39).
The essential difference between
the two laws consists of the fact that, although Vavilov clearly
noted and carefully studied the parallel variations, he did not comment
on the finite nature of the variations, the clearly defined spectra
of mutants themselves, which constitutes the inmost nature or substance
of the law of recurrent variation. Moreover, not all variation is
parallel variation − especially so in distant plant and animal
families and orders. However, in any case, the law of recurrent variation
applies as formulated above. Repercussions for the synthetic theory
of evolution
Since the foundations of the earlier “overoptimism” for
a revolution in plant breeding due to induced mutations rested on
the pillars of the synthetic theory of evolution (neo-Darwinism) − insisting,
as pointed out above, that mutations and selection are responsible
for the origin of all genes and proteins, all physiological processes
and anatomical structures of both the animal and plant kingdoms − the
question should be permitted whether the failure of mutation breeding
has had any repercussions on neo-Darwinism itself.
As far as the
author is aware, the protagonists of the synthetic theory have,
so far, avoided an adequate scientific discussion of this problem
(45). Nevertheless, the general non-performance or deficiency of mutation
breeding after altogether some 50 years of extensive experiments
with billions of induced mutations on several continents might point
to a fundamental problem of the present theory of evolution as to
the question whether mutations are really as productive as postulated
and still generally believed to be.
Moreover, several recent peer-reviewed
and further publications have added to the weight of this question
on the basis of extensive additional biological facts and mathematical
calculations (for the details, see 3-7, 12, 13, 19-21, 30, 35,
43-57, 61, 77, 78, 79, 80, 88, 90, 91, 95). In contrast to the
neo-Darwinians quoted in the introduction, the unanimous conclusion
of these authors - among them now again several Nobel laureates
in their relevant disciplines (Sir John Eccles (23), Karl von Frisch
(26), Ragnar Granit (29), Charles Townes (89), Eugene P. Wigner
(94), and others, see (50)) - is that mutations (in cooperation
with natural selection) constitute only a partial solution to cope
with the complex task of the origin of species and higher systematic
taxa and thus alternatives must be looked for and carefully considered. As far as the critical
authors are acquainted with plant breeding research and animal husbandry,
they argue that the relatively few positive mutation results have
been mainly due to losses of undesirable features and functions,
for example, lupines free of alkaloids, rapeseed without eruca acid,
peas with extended tendrils instead of compound leaves etc. Yet,
they emphasize the significant point that such losses of function
cannot explain the origin of all the genetic ‘raw materials’ necessary
for natural selection to generate the entire world of organisms.
Most of these researchers (including the author of the present
paper) have no problem in conceding, however, that mutations
and selection, as well as genetic drift, might essentially be
involved in microevolution, i.e. the formation of races and subspecies
as well as some higher systematic categories as species and genera,
which were originated by losses of gene functions, as for example,
the many cases of losses of flying abilities in insects and birds
on islands around the world, losses of scales in fish species
in closed lakes, losses of dispersion systems in island plants,
organ losses in cave animals etc.) (38, 43, 55). Concluding remarks
(1) Since in all cases of sufficiently
extensive mutagenesis experiments (saturation mutagenesis) a
large, but well-defined spectrum of mutant phenotypes has been realized
(asymptotically approaching the saturation line especially for
the micro-quantitative part of variation), the possibilities and
limits of mutation breeding are directly defined by the areas and
boundaries of this spectrum of mutants of the lines treated within
a species.
(2) Similar to the possibilities and limits of mutation
breeding of a line tested within a species, the genetic boundaries
of a species in its entirety are defined by the potentials and
limits of the gamut of functional alleles potentially realizable
for the redundant part of the genes and genomes of all individuals
belonging to an ‘actually or potentially interbreeding natural
population, which are reproductively isolated from other such groups’ (or,
in other words, the redundant genetical part of the genomes of all
recombinants and lines belonging to the “most inclusive reproductive
community”, i.e. to a Mendelian population). Although it is
self-evident that the genetic potential of an entire species is usually
larger than that of only one or a few line(s) within a species, the
dormant spectrum of mutant alleles in the redundant part of an entire
plant or animal species defines the correspondingly much larger but
also clearly finite boundaries of a species.
(3) In accord with the
law of recurrent variation, mutants in every species thoroughly examined
(from pea to man) − whether naturally occurring, experimentally
induced, or accidentally brought about − happen in a large,
but nevertheless limited spectrum of phenotypes with either losses
of functions or neutral deviations. Yet, in the absence of the generation
of new genes and novel gene reaction chains with entirely new functions,
mutations cannot transform an original species into an entirely new
one. This conclusion agrees with all the experiences and results
of mutation research of the 20th century taken together as well as
with the laws of probability. Thus, the law of recurrent variation
implies that genetically properly defined species have real boundaries
that cannot be abolished or transgressed by accidental mutations.
In contrast to the authors quoted in the introduction, yet in accord
with the group of researchers referred to under REPERCUSSIONS above,
the origin of the world of living organisms must be explained on
a basis different from that given by the synthetic theory of evolution.
For an additional detailed discussion of further points and possible
objections, see (see 1-9, 15, 20, 21, 23, 27, 30, 35, 38, 39,
43-57, 61, 65, 77-80, 86, 88, 90, 91, 94, 95) Acknowledgements
Figure 1
was drawn by the computer specialist Frieder Meis, Ludwigshafen,
Germany. Markus Rammerstorfer, Linz, Austria, suggested some further
references and changed the numbers accordingly. The English language
was checked by Hal Flemings, San Diego, USA. Notes
(1) Being aware
of the fact that mutation breeding proved to be useless in animals
and remembering the unanimous critical verdict on mutation breeding
in plants by all competent breeders and botanists alike, to wit that “the
results... have been rather meager in relation to the efforts expended” (as
documented above), the question may be raised how to integrate into
these overall conclusions the different impression sometimes elicited
by the FAO/IAEA Mutant Varieties Database (2004), speaking of 2,337 “officially
released varieties” due to induced mutations? Although in absolute
terms this number seems to be impressive at first sight, for a correct
assessment it has to be evaluated on the background of the following
information:
(a) The number includes ornamental plants, where losses
of gene functions (e.g. for leaf- and flower colour variation) are
even more relevant than for crop plants, (b) according to the FAO/IAEA “the
use of these mutants have been mostly local or regional”, (c)
also, the usage of these mutants was mainly temporal (as in most
culture varieties), (d) the higher the test standards and the more
thorough the testing procedures for officially approved varieties
of a country, the less the number of approved varieties due to mutation
breeding (e.g. most European countries have different standards as
compared to several African countries), (e) mutants have not been
consistently distinguished from recombinants (“cross” is
in fact given as one of the mutagenic agents), (f) in obligate outbreeders
the investigator almost always works with heterozygous lines (whether
a line is improved - in relation to the aims of a plant breeder -
by mutation or recombination, can in many cases only be definitely
ascertained by molecular investigations), (g) the problem to distinguish
recombination of given alleles from new mutations might be relevant
to a ceratin extent even in inbreeders due to the fact, that a certain
amount of cross-pollinations usually occurs in them as well (for
example in my own investigations in peas the outbreeding rate was
1-3%, so that up to 30% of the plants proved to display “contaminations”),
a fact often not duly considered in the work of mutation breeding
with such species and lines (interestingly a v.i.p. of the IAEA breeding
department once admitted this situation in a discussion with me,
but was of the opinion that this made no difference to him), (h)
such points as just enumerated may belong to the reasons for the
statement, that “the IAEA does not warrant the safety, quality,
viability ore purety (genetic or mechanical) of the material” for
international exchange, (i) the rest of truly induced mutations useful
for the plant breeder is usually due to losses of gene functions
in accord with the last paragraphs of the SYNOPSIS above, (j) Neither
the DFG (Deutsche Forschungsgemeinschaft/German Research Foundation)
nor the EU (European Union) has supported mutation breeding during
the last decades, (k) Germany’s practical plant breeders have
abandoned mutation breeding in the 1980s at the latest, which applies
for most European Countries, (l) last not least the number given
above must be seen in relation to the overall “officially released
varieties” on the same world-wide scale and over the same time
period, which probably will amount to several hundreds of thousands
of varieties (just to give a few numbers: there are 1,192 officially
acknowledged rose varieties in Germany alone - but the approval is
always given for only 10 years, so that the number of officially
released rose varieties for the last 50 yeras will be higher - ,
there are 3,200 different potato varieties, which are cultivated
in over 100 countries (2003), and there are about 7,500 cultivated
apple varieties.
(2) In biology the term “law” is often
interchangeably used with the label “rule”, as in the
case of the Mendelian “rules” or “laws”.
Strictly speaking, a law makes testable predictions on the basis
of a set of preconditions and does not permit any exceptions from
its deductions. Since so far I do not know of any valid exceptions
of this principle for induced and spontaneous random mutations as
deduced above, I presently prefer to speak of the “law” of
recurrent variation sensu stricto (researchers should, perhaps, constantly
remind themselves that not only mutation breeding but also any expectations
to artificially ‘speed up evolution’ (Muller) by mutations
in the wild largely failed because of this law). Yet, borderline-cases
could possibly consist of complex DNA rearrangements leading to unusual
losses of genetic functions and correspondingly rare morphological
aberrations with a low probability to independently occur again,
e. g. Tunicate in maize (Thomas Münster, MPIZ, Cologne, personal
communication), or the polymorphism conveying powdery mildew resistance
in barley (Pifanelli et al., Nature 430: 887-891, 2004). I suggest
that such cases should be included in the law as rare “borderline-cases” at
the more distal part of the saturation curve for phenotypes, inasmuch
as the barley example confers a similar phenotype to the other mlo
loss of function alleles (apart from the reversion-rate of 0.5-1
x 10-4 and a “low level growth of sporulating Bgh colonies” in
the mlo-11 plants). Moreover, it should perhaps also be considered
that in both cases further independent occurrences cannot be excluded
in the billions of plants grown each year world-wide in both species:
the world barley production was some 155 million tons in 2004 consisting
of about 3.8 x 1015 individual gains (one grain ca. 0.04 g). Hence,
the occurrence of a grain carrying a mutant gene with the low probability
of only 10-12 per gene per generation due to a spontaneous mutation
will still amount to several thousands of independent occurrences
(and thus grains carrying the mutant gene) worldwide. The number
of mutant plants will be smaller, of course, but still amounts to
several dozens and several hundreds in a hundred years. A similar
calculation can be made for the 705 million tons of maize also produced
in 2004 (one grain ca. 0.2 g). The law, however, clearly excludes
the origin of new complex functional sequences (entirely new genes
and new gene reaction chains for novel synorganized anatomical structures
and/or physiological functions) by random mutations. As for the probability
of the origin of new functional genes see and (19)-(21), (77), (78),
and (95).
References
1. Becker, H.-A., and Lönnig, W.-E. 2002,
Nature Encyclopedia of Life Sciences, Nature Publishing Group, London,
18, 559-539. 2. Becker, H.-A., Saedler, H., and Lönnig W.-E.
2002, Encyclopedia of Genetics, S. Brenner and J.H.Miller (Eds.)
Academic Press, San Diego, 4, 2020-2033. 3. Behe, M.. J. 1996, Darwin’s
Black Box, The Free Press, New York. 4. Behe, M.. J.2002, Natural
History 111, 74. 5. Behe, M. .J. 2004, Debating Design: From Darwin
to DNA, W. A. Dembski, M. Ruse (Eds.), Cambridge University Press,
Cambridge, 352-370. 6. Behe, M.. J. 2005, Design for Living. The
New York Times. 7 February 2005, Editorial Desk, p. 21. 7. Behe,
M. J. and Snoke, D. W. 2004, Simulating evolution by gene duplication
of protein features that require multiple amino acid residues. Protein
Sci. 13, 2651-2664. 8. Bennett, M. D., and Leitch I.J. 1995, Ann.
Bot. 76,113-176.
9. Bennett, M. D., and Leitch I.J. 1997, Ann. Bot.
80,169-196. 10. Bennetzen J.L. 1998, Curr. Opin. Plant. Biol. 1,
103-108.
11. Bennetzen, J.L. 2000, Plant Mol. Biol. 42: 251-269.
12. Berlinski, D. 2003, Commentary 115: 29-37 (4 April 2003). 13.
Berlinski, D. 2003, Commentary 115 (for the full discussion see David
Berlinski’s articles under: http://www.discovery.org/csc/fellows.php)
(8 July 2003) 14. Blixt, S. 1972, Agri Hort. Genet. 30, l-293. 15.
Campbell J.A., Meyer. and S. C. (Eds) 2003, Darwinism, Design, and
Public Education, Michigan State University Press, East Lansing.
16. Caspersson, T. 1946, The Nobel Prize in Physiology or Medicine
1946 Presentation Speech. From: Nobel Lectures, Physiology or Medicine
1942-1962, Elsevier Publishing Company, Amsterdam. 17. Darwin C.R.
1859, On the Origin of the Species, John Murray, London. 18. Dawkins,
R. 1995. River out of Eden. Basic Books, New York. 19. Dembski, W.
A. 1998, The Design Inference: Eliminating Chance Through Small Probabilities,
Cambridge University Press, Cambridge. 20. Dembski, W.A. 2002, No
Free Lunch: Why Specified Complexity Cannot Be Purchased without
Intelligence, Rowman & Littlefield, Lanham.
21. Dembski, W.A.
2004, The Design Revolution, InterVarsity Press, Downers Grove. 22.
Dobzhansky, T. 1937, Genetics and the Origin of Species, Columbia
University Press, New York 23. Eccles, J.C. 1992, Cosmos, Bios, Theos,
160-164. Margenau, H, and Varghese, R. A. (Eds.), Open Court, Chicago
and La Salle, Illinois. 24. FAO/IAEA, 2004, http://www-mvd.iaea.org/MVD/default.htm
25. Fischbeck, G., Röbbelen, G., Stutzer, D. 1987, Landwirtschaftliche
Pflanzenzüchtung in Deutschland. Herausgegeben vom Bundesverband
Deutscher Pflanzenzüchter e.V. Bonn. Gelsenkirchen-Buer. 26.
Frisch, K. von, 1977, Scientific Interview, Third TV-Programme, FRG,
15. 9. 1977. 27. Gene, M. 2001, Randomly Generated Biologically Functional
Proteins? http://www.idthink.net/biot/ranprot/index.html 28. Gottschalk,
W. 1989, Allgemeine Genetik. 3. Auflage, Georg Thieme Verlag Stuttgart.
29. Granit, R. 1992, Cosmos, Bios, Theos, 177-178. Margenau, H, and
Varghese, R. A. (Eds.), Open Court, Chicago and La Salle, Illinois.
30. Grasse, P.-P. 1977, Evolution of Living Organism. Academic Press,
New York, N.Y.
31. Haecker, V. 1925, Pluripotenzerscheinungen. Gustav
Fischer Verlag, Jena. 32. Hartl, D. L. 1988, A Primer in Population
Genetics. Second Edition. Sunderland/Mass. 33. Hartl, D. L. and Jones,
E. W. 1998, Genetics. Jones & Bartlett Publishing, Sudbury, Boston.
34. Hockett, E. A., and Nilan, R.A.1985, Rasmusson, D.C. (Ed.), Barley.
Madison, 187-230. 35. Junker, R. and Scherer, S. 2001, Evolution
- Ein kritisches Lehrbuch. Weyel Lehrmittel Verlag, Gießen.
36. Keefe A.D., Szostak J.W. 2001, Nature 410, 715-718. 37. Kuckuck,
H. and Mudra, A. 1950, Lehrbuch der allgemeinen Pflanzenzüchtung.
Hirzel Verlag, Stuttgart.
38. Kunze, R., Saedler, H., and Lönnig, W.-E. 1997, Adv. Bot.
Res. 27, 331-470. 39. Lamprecht, H. 1974, Monographie der Gattung
Pisum. Steiermärkische Landesdruckerei, Graz. 40. Leibenguth,
F. 1982, Züchtungsgenetik. Gustav Fischer Verlag, Stuttgart.
41. Lenski, R.E., Ofria, C., Pennock, R.T., and Adami, C. 2003,
Nature 423, 139-144. 42. Linskens, H.F. 1982, Review of: Genes, Radiation
and Society - Book by E.A.Carlson. Theor. Appl. Genet. 63, 200. 43.
Lönnig, W.-E. 1993, Artbegriff, Evolution und Schöpfung,
Naturwissenschaftlicher Verlag, Köln (and Internet edition 2002).
44. Lönnig, W.-E. 2001, The Corsini Encyclopedia of Psychology
and Behavioral Sciences, Craighead, W.E., and Nemeroff. C.B. (Eds),
John Wiley & Sons. New York, 1008-1016 45. Lönnig W.-E.
2002, Das Gesetz der rekurrenten Variation, Naturwissenschaftlicher
Verlag, Köln. (See also 1995: Streifall Evolution, Mey, J.,
Schmidt, R., Zibulla, S. (Eds.), 149-169.) 46. Lönnig W.-E.
2002, Evolution durch Genduplikationen? Naturwissenschaftlicher Verlag,
Köln. 47. Lönnig, W.-E. 2002, Coryanthes und Catasetum,
Naturwissenschaftlicher Verlag, Köln. 48. Lönnig, W.-E.
2003, Johann Gregor Mendel: Why his discoveries were ignored for
35 (72) years, Naturwissenschaftlicher Verlag, Köln. 49. Lönnig,
W.-E. 2004, Dynamical Genetics. Parisi, V., De Fonzo, V., Aluffi-Pentini,
F. (Eds). Research Signpost, Trivandrum, India. 50. Lönnig,
W.-E. 2005, Nobelpreisträger pro Intelligent Design und/oder
einen "religious impulse" in den Naturwissenschaften. Naturwissenschaftlicher
Verlag, Köln.
51. Lönnig, W.-E., and Becker, H.-A. 2004,
Nature Encyclopedia of Life Sciences, Nature Publishing Group, London.
52. Lönnig, W.-E., and Becker, H.-A. 2004, The Concise Corsini
Encyclopedia of Psychology and Behavioral Science, Craighead, W.E.,
and Nemeroff. C.B. (Eds), Wiley-VHC, New York, Vol. 3, 1008-1016.
53. Lönnig, W.-E., and Huijser, P. 1994, Plant molecular biology
manual. Gelvin, S. B., and Schilperoort, R. A. (Eds.), Kluver Academic
Publishers, Dordrecht, K1, 1-15. 54. Lönnig, W.-E., and Saedler,
H. 1994, Mol. Gen. Genet. 245, 636-643. 55. Lönnig, W.-E., and
Saedler, H. 1997, Gene 205, 245-253. 56. Lönnig, W.-E., and
Saedler, H. 2002, Annu Rev. Genet. 36, 389-410 57. Lönnig, W.-E.,
Saedler, H., Kim, J.H. 2005 (in press). 58. Lundqvist, U. 1986, Svalöf
1886-1986, Research and Results in Plant Breeding. G. Olsson (Ed.),
Stockholm, 76-84. 59. Lundqvist, U. Personal communication 1987.
60. Lundqvist, U. Personal communication; letter including all the
details 1988.
61. Margulis, L., Sagan, D. 1997, Slanted Truths: Essays
on Gaia, Symbiosis, and Evolution. Springer-Verlag, Berlin. 62.
Mayr, E. 1970, Populations, Species, and Evolution, The Belknap Press
of Harvard University Press, Cambridge, Mass. 63. Mayr, E. 2000,
Scie. Am., 283 (July 2000), 66-71. 64. Mayr, E. 2002, Laborjournal
5/2002, 26-30.
65. Meyer, S. C. 2004, Proc. Biol. Soc. Washington 117, 213-239.
66. Micke, A. 1970, Genetica Agraria 22, 262-268. 67. Micke, A. 1976,
Induced mutations in cross-breeding. IAEA (International Atomic Energy
Agency), 1 - 4. Wien. 68. Micke, A. and Weindl, K. 1983, Mutation
Breeding Newsletter 2l , 1. 69. Monod, J. 1971, Chance and Necessity.
An Essay on the Natural Philosophy of Modern Biology. Knopf. New
York. 70. Muller, H .J. 1928, Science 66, 84-87.
71. Muller, H .J.
1946, The production of mutations, Nobel Lecture. From: Nobel Lectures,
Physiology or Medicine 1942-1962, Elsevier Publishing Company, Amsterdam.
72. Murray, M.G., Peters, D.L., Thompson, W.F. 1981, J. Mol. Evol.
17, 31-42. 73. Nilsson, D.E., Pilger, S. 1994, Proc. Roy. Soc. London
B 256, 53-58. 74. Nouzová, M., Kubaláková, M.,
Zelová, M.D., Koblítzková, A., Neumann, P.,
Dolezel, J., Macas, J. 1999, Ann. Bot. 83, 535-541. 75. Nouzová,
M., Neumann, P., Navrátilová, A., Galbraith, D.W.,
Macas, J. 2000, Plant Mol.Biol. 45, 229-244. 76. Poehlmann, J. M.
1987, Breeding Field Crops. Third Edition. Van Nostrand Reinhold.
New York. 77. ReMine, W.J. 1993, The Biotic Message, St. Paul Science
Publishers, Saint Paul. 78. Scherer, S. Typen des Lebens. Pascal
Verlag, Berlin. 79. Schwabe, C. 2001, The Genomic Potential Hypothesis:
A Chemist’s View of the Origins, Evolution and Unfolding of
Life. Landes Bioscience. Georgetown, Texas. 80. Schwabe, C. 2002,
Anat. Rec. 268, 171-179.
81. Schwarz-Sommer, Z., de Andrade Silva,
E., Berndtgen , R., Lönnig, W.-E., Müller, A., Nindl, I.,
Stüber, K., Wunder, J., Saedler, H., Gübitz, T., Borking,
A., Golz, J.F.; Ritter, E., Hudson A. 2003, Genetics 163: 699-710.
82. Sengbusch, P. von 1989, Botanik. Blackwell, Hamburg. 83. Sengbusch,
R. von 1980, Von der Wildplanze zur Kulturpflanze. Published by author
(no place of publication given). 84. Simmonds, N.W. 1979, Principles
of Crop Improvement, Longman, London. 85. Simmonds, N.W., and Smartt,
J. 1999, Principles of Crop Improvement, Second edition, Blackwell
Publishing, Oxford. 86. Sternberg, R. v. 2002, Ann. N.Y. Acad. Sci.
981, 154-188. 87. Stubbe, H. 1966, Genetik und Zytologie von Antirrhinum
L. sect. Antirrhinum. VEB Gustav Fischer Verlag, Jena. 88. Swift,
D. 2002, Evolution under the Microscope, Leighton Academic Press,
Stirling University Innovation Park. 89. Townes, C. H. 1992, Cosmos,
Bios, Theos, 122-124. Margenau, H, and Varghese, R. A. (Eds.), Open
Court, Chicago and La Salle, Illinois. 90. Truman, R. 2004, Evaluation
of neo-Darwinian Theory with Avida Simualtions? PCID 3.1.1. part
1. http://www.iscid.org/papers/Truman_ComplexFeatures1_070104.pdf
91. Truman, R. 2004, Evaluation of neo-Darwinian Theory with Avida
Simualtions? PCID 3.1.1. part 2. http://www.iscid.org/papers/Truman_ComplexFeatures2_070104.pdf
92. Vavilov, N. I. 1922, J. Genet. 12, 47-89. 93. Vavilov, N. I.
1935, Chron. Bot. 13, 55-94.
94. Wigner, E. P. 1992, Cosmos, Bios, Theos, 131-132, 270-278. Margenau,
H, and Varghese, R. A. (Eds.), Open Court, Chicago and La Salle,
Illinois. 95. Wittlich, K. 1991, Über die Wahrscheinlichkeit
der zufälligen Entstehung brauchbarer DNA-Ketten. In: Zehn Paradebeispiele
gegen Zufalls-Evolution (W.-E. Lönnig, ed.), 28-39, Naturwissenschaftlicher
Verlag Köln, Cologne.
The text was slighty corrected on 1 Dezember 2007.
|